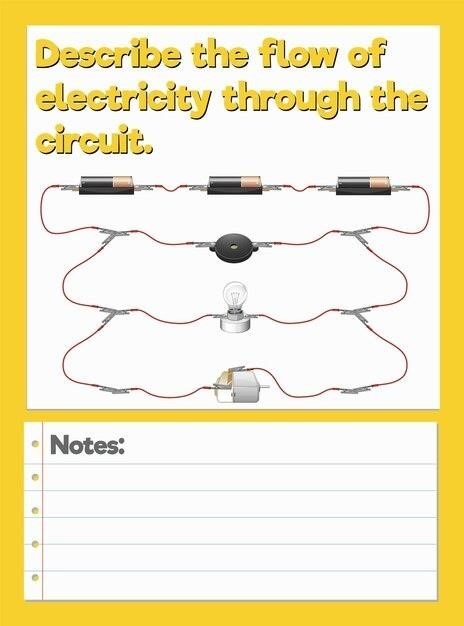
Electron Configuration Basics
Electron configuration describes the arrangement of electrons within an atom’s orbitals․ Understanding this arrangement is crucial for predicting chemical behavior․ Numerous online resources, including PDFs, offer practice problems and solutions․
Understanding Electron Configuration Notation
Electron configuration notation uses a concise system to represent the arrangement of electrons in an atom’s orbitals․ It follows a specific format, typically listing the principal energy level (n), the subshell (s, p, d, or f), and the number of electrons in each subshell as a superscript․ For example, the electron configuration of oxygen (atomic number 8) is written as 1s²2s²2p⁴․ This indicates two electrons in the 1s orbital, two in the 2s orbital, and four in the 2p orbitals․ Understanding this notation is fundamental to solving electron configuration problems and interpreting the results found in various online resources, including PDFs containing practice problems and solutions․ Mastering this notation allows you to accurately predict an element’s chemical properties based on its electron arrangement․ Many online sources provide practice exercises to help solidify this understanding;
Rules for Filling Orbitals (Aufbau Principle, Hund’s Rule, Pauli Exclusion Principle)
Several fundamental rules govern electron placement within orbitals․ The Aufbau principle dictates that electrons initially fill lower-energy orbitals before occupying higher-energy ones․ Hund’s rule states that electrons individually occupy each orbital within a subshell before pairing up․ Finally, the Pauli exclusion principle asserts that a maximum of two electrons can reside in a single orbital, and these electrons must possess opposite spins․ These principles are essential for correctly predicting electron configurations․ Numerous online resources, including PDFs containing practice questions and answers, illustrate the application of these rules․ Understanding these rules is critical for accurately determining electron configurations and subsequently predicting an element’s chemical reactivity and other properties․ Many online practice problems test your knowledge of these rules․
Practice Problems⁚ Full Electron Configurations
Mastering electron configuration requires practice․ Numerous online resources, including PDFs, provide ample problems for developing proficiency in writing full electron configurations for various elements․
Examples for Common Elements (e․g․, Na, Cl, K)
Let’s start with straightforward examples․ Sodium (Na), with an atomic number of 11, has an electron configuration of 1s²2s²2p⁶3s¹․ Chlorine (Cl), atomic number 17, is 1s²2s²2p⁶3s²3p⁵․ Potassium (K), atomic number 19, follows the pattern⁚ 1s²2s²2p⁶3s²3p⁶4s¹․ These examples illustrate how electrons fill orbitals according to the Aufbau principle, Hund’s rule, and the Pauli exclusion principle․ Many online resources, including readily available PDFs, offer detailed explanations and numerous additional examples to aid in understanding and practicing writing full electron configurations․ These resources often include worked solutions, allowing for self-assessment and improved comprehension․ Practicing with these common elements builds a strong foundation for tackling more complex configurations․
More Complex Examples (Transition Metals, Lanthanides)
Transition metals and lanthanides present a greater challenge due to the involvement of d and f orbitals․ For instance, iron (Fe), a transition metal with atomic number 26, has a configuration of [Ar] 3d⁶4s²․ Note the filling order deviates from a simple Aufbau prediction․ Lanthanides, with their filling of the 4f subshell, further complicate matters; Consider cerium (Ce), atomic number 58⁚ [Xe] 4f¹5d¹6s²․ The electron configurations of these elements often require a deeper understanding of orbital energy levels and electron-electron interactions․ Many online resources, including PDFs containing practice problems and answer keys, are available to help you master these complexities․ These resources typically provide step-by-step solutions to aid in understanding the nuances of electron filling in transition metals and lanthanides, reinforcing the principles involved․
Practice Problems⁚ Abbreviated Electron Configurations
Using noble gas notation simplifies electron configurations․ Practice writing abbreviated configurations for various elements to enhance understanding and proficiency․ Many online resources offer such practice problems with solutions․
Noble Gas Notation Examples
Noble gas notation streamlines electron configuration representation․ Instead of writing the full electron configuration, you can abbreviate it using the symbol of the preceding noble gas in brackets, followed by the remaining electron configuration․ For example, sodium (Na) has an electron configuration of 1s²2s²2p⁶3s¹․ Using noble gas notation, we can simplify this to [Ne]3s¹, where [Ne] represents the electron configuration of neon (1s²2s²2p⁶)․ This method is particularly useful for elements with many electrons, making the configuration easier to read and understand․ Numerous online resources, including PDFs, provide numerous examples and practice problems using noble gas notation, allowing you to refine your skills and comprehension of electron configurations․ These resources often include detailed explanations and step-by-step solutions, making learning more efficient and effective․ Mastering noble gas notation is essential for deeper understanding of atomic structure and chemical properties․
Advanced Abbreviated Configuration Practice
Beyond basic noble gas notation, advanced abbreviated configurations tackle more complex elements, including transition metals and lanthanides/actinides․ These elements involve partially filled d and f orbitals, requiring a more nuanced understanding of electron filling rules (Aufbau principle, Hund’s rule, Pauli exclusion principle)․ Practice problems often involve identifying the correct abbreviated configuration for these elements, and then using this to determine the number of valence electrons or unpaired electrons․ Many online resources, such as PDFs and interactive exercises, provide extensive practice with these more challenging elements․ These resources often incorporate diverse question types, such as matching configurations to elements, identifying incorrect configurations, and predicting magnetic properties based on the electron configuration․ Successfully tackling these advanced problems demonstrates a solid grasp of electron configuration principles and their application to complex atoms․
Identifying Valence Electrons
Valence electrons, crucial for chemical bonding, are readily identified from an atom’s electron configuration․ Practice problems often focus on this skill․
Determining Valence Electrons from Electron Configurations
Valence electrons, the outermost electrons in an atom, are key to understanding an element’s reactivity and bonding behavior․ Determining them from electron configurations involves identifying the electrons in the highest principal energy level (n)․ For main group elements, this is straightforward; the valence electrons are those in the s and p orbitals of the highest energy level․ However, transition metals present a slightly more complex scenario, as they may involve d orbitals as well․ Practice problems often involve identifying valence electrons for various elements, strengthening understanding of their chemical properties․ Many online resources provide numerous examples and exercises to master this crucial skill․ These resources often include detailed explanations and step-by-step solutions, enhancing the learning process․ The ability to accurately determine valence electrons from electron configurations is essential for understanding chemical bonding and predicting reaction outcomes․
Relating Valence Electrons to Chemical Properties
Valence electrons directly influence an element’s chemical behavior․ The number of valence electrons determines an element’s reactivity and the types of bonds it can form․ Elements with similar valence electron configurations often exhibit similar chemical properties, as seen in periodic trends․ For instance, elements in the same group (column) on the periodic table have the same number of valence electrons and therefore share similar chemical characteristics․ Understanding this relationship is fundamental to predicting how elements will react with each other․ Practice problems often focus on connecting the number of valence electrons to the element’s group on the periodic table and predicting its bonding behavior; Online resources, such as PDFs containing questions and answers, prove invaluable for reinforcing this crucial connection between electron configuration, valence electrons, and chemical properties․ Mastering this concept is essential for comprehending a vast array of chemical phenomena․
Applications of Electron Configuration
Electron configurations predict ion formation, magnetic properties, and bonding behaviors․ Practice problems help solidify understanding and application․
Predicting Ion Formation
Understanding electron configuration is key to predicting how ions form․ Atoms tend to gain or lose electrons to achieve a stable electron configuration, often resembling a noble gas․ For example, sodium (Na) readily loses one electron to achieve the stable neon configuration, forming a +1 ion (Na+)․ Chlorine (Cl), conversely, gains one electron to achieve the stable argon configuration, forming a -1 ion (Cl-)․ This electron transfer is fundamental in ionic bonding․ Practice problems involving electron configurations help students master predicting the charge of ions formed by various elements․ The ability to predict ion formation is crucial for understanding chemical reactions and compound formation․ Mastering this concept enhances problem-solving skills in chemistry․
Understanding Magnetic Properties
Electron configuration directly relates to an element’s magnetic properties․ Paramagnetic substances, such as many transition metals, possess unpaired electrons in their electron configuration, leading to attraction towards a magnetic field․ Conversely, diamagnetic substances, with all electrons paired in their configurations, exhibit a weak repulsion from a magnetic field․ The presence or absence of unpaired electrons, readily determined from an electron configuration diagram (e․g․, orbital box diagrams), dictates the magnetic behavior․ Practice questions often involve determining the magnetic nature of an element based solely on its electron configuration․ This connection between electronic structure and macroscopic properties is a key concept in chemistry and materials science․